By Dr. Hojun Yoon, Yoav Banin

CPV and Triple Junction (3J) CPV Cell Characteristics
All PV technologies aim to minimize the cost of electricity generated from their systems. CPV aims to achieve this by minimizing the use of expensive solar cell materials, through using less expensive materials to collect and deliver the sunlight to the solar cell. Basic elements of a CPV system include optics [optics can further be classified into two categories: (1) primary optics being either refractive or reflective and (2) concentration method being either point focus or dense array. With the refractive point focus approach becoming the dominant choice, this article addresses the topic using them as a reference point], tracking (2-axis), high-efficiency solar cells, and thermal management. Using optics, sunlight is concentrated (typically by a factor of ~500-1000X in the case of HCPV) onto a small high-efficiency solar cell, typically 0.3-1.0 cm2 in size. Because CPV uses only the Direct Normal Incidence (DNI) sunlight, tracking is required to precisely follow the sun at all times. Although this is an extra cost item, it enables ~30-40% higher electric energy generation compared to fixed flat-plate PV panels. Despite the optical loss associated with concentrating the sunlight, the near 40% efficiency of Triple Junction (3J) CPV solar cells enables module DC efficiencies over 30% and AC system efficiencies over 25% with plenty of room for future improvements, unlike other PV technologies.

The remainder of this section provides a brief primer on 3J CPV cell characteristics, relevant for the main focus of this article. Specifically, the basic design and its impact on the current distribution among the three junctions are described. Numerous references are readily available in the literature related to this III-V cell technology, initially developed for and primarily used in space applications2). Here, in Figure 1, a basic comparison is shown between a ty pical 3J CPV cell and a conventional Si cell, highlighting the more efficient utilization of the solar spectrum by the 3J cell. The three junctions, often referred to as top, middle, and bottom, are typically made using GaInP, GaInAs, and Ge, respectively, which have sequentially lower bandgaps (~1.85 eV, ~1.4 eV, 0.67 eV) enabling appropriate light absorption and transmission. It also depicts the series-connected nature of the 3J cell, which limits the device Isc to the smallest current producing junction. This last point is important to understand, as there are many factors that influence the current output of the individual junctions: the natural variation in the sunlight itself, the design of the optics, and the variation of the spectral response of the cell itself.
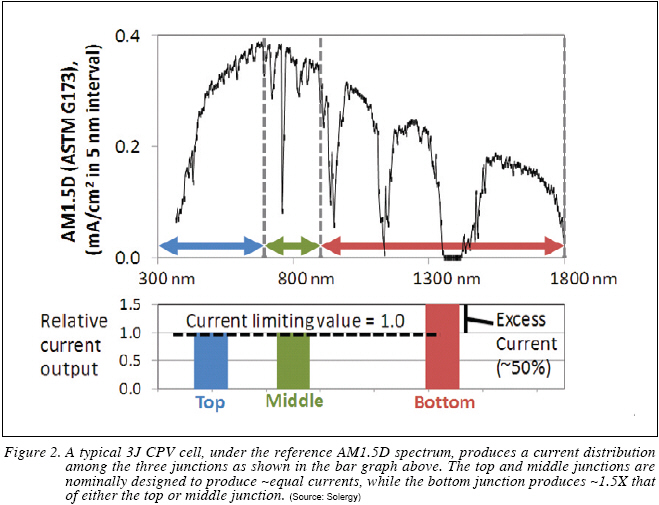
A nominal 3J CPV cell is designed such that under the reference AM1.5D spectrum (ASTM G173), the top and middle junctions produce roughly equal amount of current, while the bottom junction produces an excess, typically about 50%, or 1.5X that of the top or the middle junction. This is shown in the bar graph in Figure 2, where a normalized value of 1 is assigned for the top and middle junctions (note: a typical current density of 14 mA/cm2 is obtained under AM1.5D, 1-sun, 1000 W/m2). In the next section, analyses are made with reference to this normalized value of 1 as a way to quantify the effective optical efficiency of the primary optics.
Limitations of Widely Used Primary Optics (PMMA)
The predominant optical design used by CPV companies today is based on refractive Fresnel optics, made using Polymethylmethacrylate (PMMA) or Silicone-On-Glass (SOG), which has been introduced more recently. Neither is a true all-glass design, which raises the question of long-term reliability, a prerequisite for all PV systems. For example, studies have highlighted the degradation of the optical and mechanical properties of PMMA3), which would clearly shorten the useful lifetime of the product. And while the SOG design enjoys the benefit of glass as the front surface, Fresnel structures are made using silicone and attached to the glass underside; this hybrid structure combines two materials with very different thermal expansion properties, posing serious challenges for maintaining high mechanical stability (e.g., adhesion, which would directly impact optical efficiency). Although SOG may demonstrate high performance initially, as this is a newly introduced technology, not much is known about its field performance over an extended period of time. Reports discussing some of these issues have been presented at a recent conference.4)
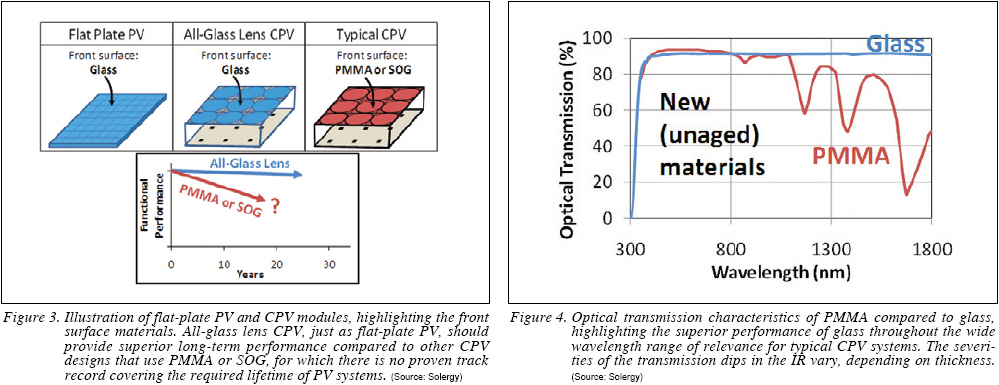
Figure 3 depicts flat-plate PV, typical CPV and all-glass lens CPV modules, highlighting the front surface materials used in each. For CPV, the front surface material must serve a dual role: protection of the module and concentration of the sunlight. All-glass lens CPV enjoys the proven durability offered by conventional flat plate modules, which use glass as the front surface. The schematic plot shown in the figure describes the inherent durability associated with an all-glass component compared to the uncertainty associated with PMMA or SOG, for which there is no proven track record covering the required lifetime of PV systems.
Figure 4 compares the optical transmission characteristics of typical PMMA (5mm) compared to a glass sample (10mm), which was cut out directly from an actual CPV glass lens. While the glass has a high, flat-transmission profile throughout the entire wavelength range of interest, the PMMA, although it displays high transmission in the visible region, exhibits transmission dips in the IR, the severity of which depends on the thickness.
The optical loss incurred by a new PMMA lens as it relates to the 3J CPV cell is shown in Figure 5. As an example, the PMMA transmission curve shown in Figure 4, was applied to the AM1.5D spectrum, with the reduction/modification indicated by the colored regions. One noteworthy point here is the reduction in the available light for the bottom junction: the excess current is now reduced to ~30%, compared to ~50% for the case of unmodified spectrum. The same analysis performed for glass, as can be deduced from the flat transmission curve of glass in Figure 4, results in an unchanged amount of excess current (i.e., retains the ~50% excess current) for the bottom junction. Fortunately for PMMA, even with this reduction, the bottom junction still enjoys excess current such that it usually has negligible impact to the overall 3J device performance. In fact, the effective optical efficiency as represented by the current limiting value in the bar graph is ~92%, limited only by reflection losses. However, this non-flat transmission profile of PMMA is expected to be problematic for future multijunction device designs, where, for example, the Ge junction is replaced with a higher bandgap material. In such cases, the current balancing among the multiple junctions becomes highly important for producing high efficiency devices.
Although PMMA lenses may provide adequate performance during the early stage of field operation, long-term reliability performance cannot be guaranteed. A detailed study on the durability of PMMA lenses used in CPV has been initiated at NREL, and has pointed out some of the reliability issues including yellowing, permanent soiling, and mechanical cracking/hazing/warping, all of which directly or indirectly impact the optical performance of the PMMA. Other inferior properties such as high water absorption, low chemical stability, and low mechanical strength compared to glass, make it extremely challenging for PMMA to maintain high performance over a long period of time under harsh outdoor conditions. Here, we select as an example the yellowing/darkening phenomenon of PMMA as it relates to optical transmission loss and its corresponding impact to the 3J CPV cell performance.

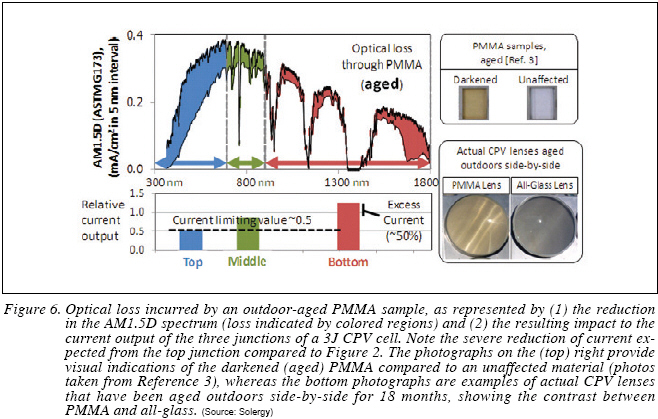
An example case of severe yellowing of an aged PMMA sample is taken from the NREL study and examined in Figure 6. Although this is an extreme case, it illustrates the detrimental effect of outdoor exposure on the optical transmission of PMMA. In this example, there is severe optical loss across the top junction region, resulting in an effective optical efficiency of about 50% (referenced to Figure 2 baseline case). Optical photographs showing the corresponding visual yellowing of this sample, compared to an unaffected sample, are also shown in the figure (top right photos from Reference 3). As a further example, the bottom right photographs compare actual CPV lenses (PMMA vs. all-glass) that have been aged outdoors side-by-side for 18 months, revealing the similar yellowing of the PMMA lens.
CPV Performance Using All-Glass Primary Lens
Unlike any optical design employed by CPV system manufacturers, Solergy, Inc.¡¯s primary optics are based on a true all-glass refractive lens, providing assurance of long term reliability and performance. One of the main reasons why true all-glass refractive lenses are not utilized for CPV primary optics is due to the lack of a cost-effective process for manufacturing precise, large-dimension, high optical efficiency lenses. Because cost-effective CPV systems require concentration factors that are in the range 500X-1000X, practical primary optics require dimensions that range from 20 cm2 to 1000 cm2 for cell sizes that range from 4 mm2 to 100 mm2. With many of the CPV systems using cell sizes of ~30 mm2 or greater, this translates to individual primary optics dimensions of greater than 10 cm length (side of a square or diameter of a circle), and exceeding 30 cm length for 100 mm2 cells. Fabricating optical quality glass lenses of these dimensions cost effectively is not a trivial task, but would represent a solution that eliminates the reliability and performance risks associated with the type of materials used for CPV primary optics.
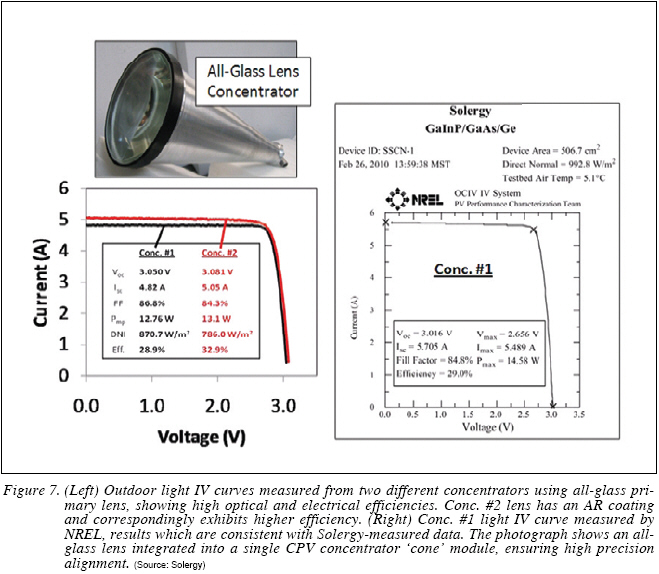
Solergy has successfully developed a proprietary manufacturing method to produce such optical quality, large-area, all-glass plano-convex lenses. Both the optical design of the lense, which provides for a uniform distribution of radiation intensity on the surface of the cell and corrects all chromatic aberration effects, and the fabrication method are patent-pending. Although heavier than a Fresnel lens design, a plano-convex lens assures higher optical efficiency (e.g., no draft loss) and mechanical strength, and lenses of >25 cm in diameter with highly precise curved surfaces are produced from a mold that do not require subsequent working of the curved surfaces. Furthermore, this lens design--without any fine Fresnel ¡®teeth¡¯ structures--offers various options for applying cost-effective Anti-Reflective (AR) coatings.
To demonstrate the high optical and electrical performance achievable from CPV modules using all-glass primary lenses, Figure 7 shows outdoor light IV curves measured from two different concentrators, Conc. #1 and Conc. #2, with 28.9% and 32.9% efficiency, respectively. Each concentrator is a single lens-cell pair unit consisting of an all-glass primary lens that provides ~500X geometric concentration onto a 1 cm2 cell. The higher performance of Conc. #2 is primarily due to the use of AR coating on the glass lens as well as a higher efficiency 3J cell. The light IV curve (of Conc. #1) on the right side of the figure showing 29.0% efficiency was measured by NREL, results which are consistent with internally measured data. While these results are from single lens-cell pair units, larger module units (> 600 W) are achieving over 28% efficiency.
In addition to the all-glass lens, Solergy has introduced a unique product architecture based on a cone-shaped basic power generation unit that incorporates a single lens-cell pair. Unlike the box-shaped modules shown in Figure 3, the cone concentrator provides a direct physical coupling between lens and cell. This guarantees precise alignment both during assembly and outdoor operation over time. The box module, on the other hand, can suffer from misalignment between some of the lens-cell pairs due to assembly errors, thermal expansion mismatch between the top and bottom of the box, and subsequent structural deformation during outdoor operation.

Figure 8 further highlights the high optical and electrical efficiencies obtained using all-glass primary lenses. Isc vs. DNI (W/m2) is plotted using measured light IV data from the two concentrators shown in Figure 7, Conc. #1 and Conc. #2. Multiple datapoints are plotted showing the nominal linear behavior of the Isc vs. DNI. As a way to characterize the total optical efficiency of the concentrator, two reference lines are plotted that represent 100% and 80% optical efficiency. (Note: Although these optical efficiencies should not be taken as absolute values--due to the assumptions noted--they are still useful as a semi-quantitative comparison tool.) The 100% line represents the hypothetical Isc that would be expected if all the DNI that is incident on the primary optics aperture area were transferred to the cell (i.e., there is zero optical loss). The 80% line represents the expected Isc of the concentrator assuming a 20% total optical loss. As can be seen, the datapoints from Conc. #1 with ~29% efficiency align closely with the 80% line, while Conc. #2 with 32.9% efficiency exhibits a much higher optical efficiency.
As demonstrated here, high performance, large-area, all-glass refractive lens for CPV can indeed be fabricated in a way that has a clear pathway for low-cost and high-volume manufacturing. The all-glass design has the inherent advantage of guaranteeing long-term durability, a feature that cannot be offered by PMMA or SOG lenses. While other components--tracking, solar cell, heat management--are equally important for a successful CPV system, an all-glass lens optics may hold the key to truly realizing the promise of CPV technology.
All-glass refractive lens for CPV primary optics is expected to provide the long-term reliability and performance necessary for successful CPV systems. Up until now however, no CPV system has implemented such a component, largely due to the difficulty in producing all-glass lenses (1) of large dimensions with high precision, (2) with high optical efficiency (>85%), and (3) using a low-cost manufacturing method. Alternative material such as PMMA has been widely adapted as it provides adequate combination of initial performance, cost, and weight parameters, but these benefits come at the expense of long-term reliability concerns, including but not limited to: yellowing; mechanical cracking, hazing, warping; permanent soiling--all of which directly or indirectly lead to optical transmission loss and premature degradation of the system. A first-ever demonstration of CPV modules using all-glass refractive lenses has been presented with DC module efficiency as high as 32.9%, paving the way for a truly robust, reliable, and bankable CPV system.
Dr. Hojun Yoon is Director of Solar Cell Technology at Solergy (www.solergyinc.com). Prior to joining Solergy in 2008, Dr. Yoon spent 10 years at Spectrolab, Inc. (A Boeing Company), the world¡¯s leading supplier of high-efficiency multijunction solar cells for CPV and space applications. As a Boeing Associate Technical Fellow at Spectrolab, Dr. Yoon was involved in various R&D activities of III-V multijunction solar cells, including the demonstration of the first solar cell device ever to exceed 40% efficiency. Dr. Yoon has published over 30 papers related to semiconductor materials/devices and has three patents (one pending) related to solar cells. Dr. Yoon has a Bachelor¡¯s degree in Applied Sciences in Engineering from Rutgers, The State University of New Jersey, and an M.S. and Ph.D. in Materials Science & Engineering from the University of California, Los Angeles.
Yoav Banin is Co-Founder and Chief Executive Officer of Solergy. Banin has 15 years¡¯ operating experience in leading teams that create and sell innovative high-tech products. Prior to co-founding Solergy, Banin was Director of Products at Mercury Interactive (sold to HP) where he invented and launched new enterprise software products that generated over $50M in revenue within three years. Banin holds a Bachelor¡¯s degree with High Honors in Applied Mathematics from UC Berkeley and a Master¡¯s degree in Materials Science & Engineering from Stanford University.
REFERENCES
1) Spectrolab, Inc. (www.spectrolab.com) has recently introduced a 3J CPV cell product with average efficiency of 39.2%.
2) A large number of technical papers related to III-V solar cell technology has been published over the last two decades in the Proceedings of the IEEE Photovoltaic Specialists Conference (PVSC).
3) D.C. Miller, L.M. Gedvilas, B. To, C.E. Kennedy, S.R. Kurtz, ¡®Durability of Poly (Methyl Methacrylate) Lenses Used in Concentrating Photovoltaic Modules¡¯, Proc. SPIE Optics and Photonics Conf., 7773, 7773-2, 2010.
4) 3rd International Workshop on CPV: Optics-Materials-Modules-Bankability-Grids, Bremerhaven, Germany, Oct. 2010.
For more information, please send your e-mails to pved@infothe.com.
¨Ï2011 www.interpv.net All rights reserved.